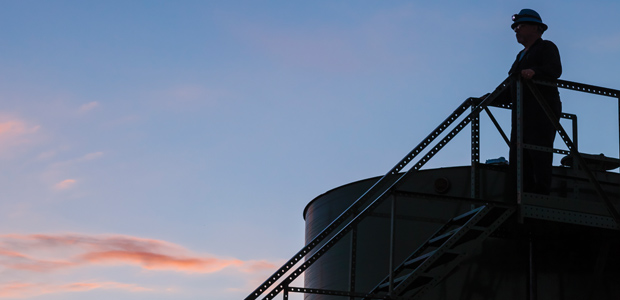
Enhanced Oil Recovery
Ethane From Shale Plays Opens New EOR Opportunity For Conventional Oil Reservoirs
By Patrick L. McGuire, Ryosuke Okuno, Thomas L. Gould and Larry W. Lake
LAKEWOOD, CO.–The United States pioneered the science of injecting carbon dioxide into conventional reservoirs to enhance oil recovery, and the Permian Basin has long been the international hub of CO2 EOR activity. CO2 EOR is a very successful and mature technology in onshore projects. The risks are low, primarily because there is no exploration uncertainty. However, the primary limiting factor to CO2 EOR is the availability of low-cost carbon dioxide.
Simply put, there is not enough cost-effective CO2 available to undertake all the potential EOR opportunities in the lower 48. With most economic sources of natural CO2 already on line, and developing substantial volumes of anthropogenic supplies difficult, finding an effective alternative to CO2 could hold tremendous value in EOR applications. The shale revolution is providing that alternative in the form of the ethane extracted from the rich gasses and volatile oils produced from unconventional reservoirs.
The abundance and affordability of ethane make it a viable option to supplement successful CO2-based EOR projects. Ethane presents a significant opportunity to add new gas injection EOR projects in mature conventional fields in oil-producing basins throughout the lower 48. Good EOR targets exist almost everywhere, given a sufficient oil price and an appropriate low-cost injecting agent.
Successful EOR projects always are based on the economical availability of an injectant. The beauty of ethane is that large volumes of inexpensive supplies have been made available by the development of shale plays, and a growing U.S. ethane infrastructure can deliver that ethane to locations where potential EOR targets are plentiful. Moreover, ethane has benefits over carbon dioxide in EOR applications, including having more solubility in oil, lower minimum miscibility pressures (MMPs), and better solvent efficiency. In addition, ethane-based EOR is operationally simpler than CO2-based projects.
The magnitude of U.S. EOR is measured in the tens of billions of barrels of additional recovery. Most of that recovery potential is concentrated in mature onshore fields in which costs are relatively small and the geology is well understood. A U.S. Department of Energy report on “next generation” CO2 EOR found that an estimated 60 billion barrels of oil could be recovered economically through gas EOR in lower-48 fields, and another 30 billion plus barrels could be recovered in onshore residual oil zone applications (2012 ARI update of DOE/NETL-2011/1504).
The vast majority of U.S. oil production from CO2 EOR comes from the Permian Basin, followed by the Gulf Coast, Rocky Mountain and Mid-Continent regions. Combined, all active U.S. projects inject about 60 million metric tons of CO2 annually (3 million cubic feet a day). The amount of new CO2 supply that would have to be injected to develop the 60 billion barrels of reserves in mature onshore fields is estimated by DOE to be on the order of 17 billion metric tons (320 trillion cubic feet).
Bridging The Gap
How can the industry bridge the huge gap between available CO2 supply and future demand to recover even a relatively small portion of those reserves with EOR gas injection projects? One answer is in Figure 1, which shows U.S. EOR production by recovery mechanism. The blue wedge is EOR from both miscible and immiscible hydrocarbon gas (non-CO2) injection, virtually all of which is located on the Alaska North Slope.
As with CO2 EOR, the engineering and economic issues and opportunities of hydrocarbon gas EOR are well understood and have been proven by decades of research and field experience. But the Alaskan EOR experience is different from the lower 48 because there has been no market for gas produced on the North Slope, and operators could take advantage of enriched gas injection primarily to recover more oil. The conventional wisdom has been that hydrocarbon gas EOR is too expensive in the lower 48, given the value of natural gas, but ethane from shale plays is changing that.
The North Slope projects have injected methane enriched with ethane, propane and butane. The Prudhoe Bay miscible gas project makes an interesting case study. It is the world’s largest enriched gas flood, with an ultimate EOR recovery expected to range between 400 million and 500 million barrels.
A typical Prudhoe Bay miscible injectant (MI) composition contains roughly 20 mole percent CO2 and 20 mole percent ethane (there are no dedicated CO2 projects in Alaska; the MI contains CO2 as part of the mix). Slim tube experiments show that the MI is miscible at the average reservoir pressure of 3,600 psi–conditions at which CO2 is clearly immiscible. In this system, CO2 is a carrier gas, and miscibility is provided by the ethane and propane.
At the North Slope West Sak Field, experimental work to investigate ethane and CO2 as EOR injectants for the viscous oil showed that gas solubility and viscosity reduction were much greater for ethane than for CO2 at similar conditions. In fact, CO2 was unable to develop dynamic miscibility with West Sak crude at reservoir pressure and temperature conditions. Ethane was miscible at 600 psi, while CO2 failed to achieve miscibility even at pressures as high as 6,600 psi. In this viscous oil system, ethane is far more effective as an EOR injectant than CO2.
Ethane’s compositional properties give it superiority over carbon dioxide as an EOR injectant in terms of solubility, swelling and viscosity reduction, as well as in terms of developing multiple-contact miscibility. Ethane has a molecular weight of 30.07 pounds per lb-mole and a critical density of 12.9 pounds per cubic foot compared with 44.01 and 29.1, respectively, for CO2. Ethane and CO2 have almost identical critical temperatures (»90 degrees Fahrenheit), while the critical pressure of CO2 is roughly 50 percent higher than ethane (1,071 versus 707 psia).
To achieve a similar liquid-like state in the reservoir, CO2 requires a substantially higher reservoir pressure. This has major implications for shallow, low-pressure reservoirs as well as for deep, hot reservoirs such as Prudhoe Bay. Ethane may have a lower density than CO2 in the reservoir, which could influence gravity override and impact vertical sweep efficiency.
Operational Issues
In addition to injectant supply, there are other challenges to implementing CO2 floods. One of the most significant operational drawbacks is the corrosive nature of CO2. Corrosion is a problem in surface facilities, pipelines, injection wells and production wells. These problems are well understood, and while successful mitigation strategies have been developed for components such as meters, wellhead trees, valves, tubing, packers and cements, the corrosiveness of CO2 impacts project cost profiles.
In contrast, ethane is noncorrosive, and by dilution, should act to reduce the partial pressure and corrosivity of any acid gas components that may be present in produced fluids. There are no foreseen additional metallurgical requirements to implement ethane water-alternating-gas (WAG) injection. However, because ethane is an excellent solvent, it will affect lube oils, greases, thread sealants, and O-ring and valve-packing elastomers in a similar manner to CO2.
As with any gas injection project, tubing collar leaks could be a problem with ethane injection. New or reconditioned tubing with premium threads and an ethane-resistant thread compound may be warranted. The advantages of ethane WAG over CO2 WAG will be particularly important in areas with limited corrosion problems where the produced oil is sweet.
An additional advantage of ethane is water solubility. CO2 is about 20 times as soluble in water as ethane. In addition to increased corrosivity, the “parasitic losses” of CO2 to the large volumes of water contacted in a mature waterflood will increase significantly the volume of CO2 required for a WAG flood.
One study of a representative reservoir showed that more than 2 Bcf of CO2 per square mile was trapped by being dissolved in the reservoir brine at a solubility of 140 cubic feet of CO2 per barrel. Ethane will not experience the solution trapping associated with CO2. As a result, there is a large economic benefit for comparable ethane EOR projects.
A final advantage of ethane over CO2 is that the produced gas from an ethane WAG flood has intrinsic value as sales gas or fuel, and no acid-gas removal plant is required either for gas sales or reinjection. High gas-to-oil ratio fields before significant ethane breakthrough could produce lean gas that was too diluted with methane to make recycling desirable. The gas could continue to be sold or burned for fuel until the gas C2+ mole fraction becomes high enough to be a good injectant. Eventually, returned ethane will overwhelm the produced solution gas.
Example Target Fields
Extensive ethane infrastructure, including ethane and natural gas liquids pipelines from the Bakken, Eagle Ford, Marcellus/Utica and other shale plays has opened ethane EOR opportunities in areas that either lack sufficient CO2 supplies, or where fields are not appropriate for CO2 WAG.
There are several types of ethane EOR targets. Evaluations of three examples–the Lawrence Field in the Illinois Basin, Cushing Field in Oklahoma, and East Texas Field–provide insights on the various types of fields that could be good candidates for ethane EOR. These evaluations focus on WAG displacement efficiency and miscibility in the reservoir, as analyzed using a commercial reservoir simulator, and are not intended to accurately represent reservoir geology or sweep efficiency.
The Illinois Basin is a very promising target for gas EOR, but no viable source of CO2 exists to develop large remaining reserves in legacy assets such as the Lawrence Field. The largest field in the basin, it was discovered in 1906 with an estimated 1.05 billion barrels of original oil in place. Cumulative oil recovery is less than 40 percent of OOIP.
One obvious target for a WAG project in the Lawrence Field is the shallow Bridgeport interval at a depth of 950 feet. Given its depth, a safe injection pressure would be perhaps no more than 700 psi.
The field is located approximately 20 miles north of Enterprise Products Partners’ ATEX ethane pipeline terminal at Princeton, In. Slim tube simulations using 100 cells in a finite difference model were run to determine the MMP of both ethane and CO2 in the Lawrence Field. The analysis used an oil composition of 34 degrees API gravity and reservoir temperatures of 71 and 80 degrees, respectively, for the shallow Bridgeport and deeper Cypress intervals. The MMP for ethane in the Bridgeport was slightly less than 600 psi, while the MMP for CO2 was 950 psi.
A quarter-five-spot model of the Bridgeport was built using the SPE3 comparative solution project problem (SPE3 solution) with 9x9x4-layer gas cycling and blow-down). Reasonable estimates of the depth, initial pressure, reservoir thickness, well spacing, fluid composition, average porosity, and average permeability from the Bridgeport were used in the model.
A 10-acre spacing base model was waterflooded for 20 years. For the ethane evaluation, the model was placed on waterflood for 4,000 days, and then 12 WAG cycles of ethane and water were injected at 700 psi. A similar procedure was followed to inject 12 WAG cycles of CO2 and then 12 cycles of methane. In these models, CO2 and ethane had the same gas-like relative permeability.
The ethane WAG ultimately recovered an additional 11.8 percent of the OOIP at a gross efficiency of 1.8 Mcf of ethane per barrel of oil recovered. The net efficiency (including gas recycle) was a very attractive 0.9 Mcf/bbl. Figure 2A shows the results of the pattern model waterflood and ethane WAG simulations, while Figure 2B shows a comparison of the ethane WAG and the CO2 WAG. The CO2 WAG recovered an additional 3.2 percent OOIP at a gross efficiency of 7.3 Mcf of CO2 per barrel. Net efficiency was 3.7 Mcf/bbl. As expected, the methane WAG recovered no additional oil.
Oil saturation and viscosity maps from the ethane and CO2 WAG runs are in Figure 3. The ethane WAG was miscible and reduced the oil viscosity by a factor of 10. The CO2 WAG was not miscible, and reduced the oil viscosity by about half.
Cushing Field
The Mid-Continent also is a very promising target for ethane EOR. Multiple CO2 floods are in operation in Oklahoma, but there are several other mature fields with more than 1 billion barrels of remaining oil in place and excellent reservoir properties for CO2 WAG.
The Cushing Field in Oklahoma was chosen to evaluate ethane EOR because it is centrally located in the “pipeline capital of the world,” with very large volumes of ethane flowing into and out of the area. Slim tube simulations using 100 cells in a finite difference model were run to determine the MMP of both ethane and CO2 at an oil composition of 38 degrees API gravity and a reservoir temperature of 108 degrees. The MMP for ethane in the Cushing Field was 875 psi, while the MMP for CO2 was 1,250 psi.
A quarter-five-spot model was built using the SPE3 solution and reasonable estimates of depth, initial pressure, reservoir thickness, well spacing, fluid composition, average porosity, and average permeability. A 20-acre spacing base model was waterflooded for 20 years. For the ethane evaluation, the model was placed on waterflood for 4,000 days, and then 16 WAG cycles of ethane and water were injected at 1,600 psi (Figure 4A). A similar procedure was followed to inject 16 WAG cycles of CO2, and then 10 cycles of methane.
The ethane WAG recovered an additional 9.1 percent of OOIP at a gross efficiency of 3.8 Mcf per barrel of oil. The net efficiency was a very attractive 1.6 Mcf/bbl. The CO2 WAG recovered an additional 6.5 percent of OOIP at a gross efficiency of 4.3 Mcf of CO2 per barrel. Net efficiency was 2.8 Mcf/bbl. The methane WAG did not recover any additional oil.
Figure 4B compares the Cushing ethane WAG and the CO2 WAG results. Oil saturation and oil viscosity maps from the ethane and CO2 WAG runs are shown in Figure 5. The ethane WAG was miscible and reduced oil viscosity by almost an order of magnitude. The CO2 WAG was not miscible away from the injection well, but significantly swelled the oil and reduced the oil viscosity by a factor of four. Both ethane and CO2 had good gross and net gas efficiencies.
East Texas Field
The supergiant East Texas Field has an OOIP of 7 billion barrels. The field was chosen for ethane EOR evaluation because it is a very large target and is connected to the ATEX ethane pipeline, with significant volumes of ethane flowing through the area.
One major issue is the large number of old wells in the field. With most wells more than 80 years old, a 2014 report concluded that the possibility of casing leaks in the aging wellbores could be a difficult problem to overcome for CO2 flooding. Increasing the average reservoir pressure in a WAG project much above the current 1,100 psi also likely would result in gas containment issues.
Slim tube simulations were again run to determine the MMP of both ethane and CO2 using an oil composition of 39 degrees API gravity and a reservoir temperature of 146 degrees. The MMP for ethane in the East Texas Field was 1,150 psi, while the MMP for CO2 was 1,900 psi. The slim tube simulation comparison with the CO2 Berea Sand core flood and slim tube experimental data was quite good.
A quarter-five-spot model was again built using the SPE3 solution and reasonable estimates of depth, initial pressure, reservoir thickness, well spacing, fluid composition, average porosity, and average permeability. Injection pressure was limited to 1,600 psi to reduce gas containment issues. A 10-acre spacing base model was waterflooded for 20 years. The model was placed on waterflood for 4,000 days, and then 12 WAG cycles of ethane and water were injected at 1,600 psi (Figure 6A). A similar procedure was followed to inject 12 WAG CO2 cycles and 12 methane cycles.
The ethane WAG recovered an additional 10.9 percent of OOIP at a gross efficiency of 7.1 Mcf of ethane per barrel. The net efficiency was a very attractive 1.5 Mcf/bbl. The CO2 WAG recovered an additional 3.0 percent of OOIP at a gross efficiency of 18.4 Mcf/bbl. Net efficiency was 3.3 Mcf/bbl. The methane WAG again did not recover significant additional oil.
Figure 6B compares the ethane and CO2 results. Figure 7 shows oil saturation and oil viscosity maps from the ethane and CO2 WAG runs. The ethane WAG was miscible and reduced oil viscosity significantly. The CO2 was near-miscible at the injection well, but did not effectively reduce the residual oil saturations at the average pattern pressure of 1,100 psi.
Table 1 shows a summary of the EOR performance, and the gross and net solvent efficiencies for the three example fields at assumed costs of $4.15 an Mcf of ethane and $2.00 an Mcf of CO2. In many locations, ethane is available for less than $1.00 an Mcf because of transportation costs to a sales hub.
As these evaluations demonstrate, ethane’s higher solubility in oil and lower MMPs allow ethane EOR to recover more oil than CO2 EOR in shallow reservoirs such as the Lawrence Field and low-pressure settings such as the East Texas Field. Both gasses work well in higher-pressure fields such as Cushing Field, allowing ethane to play a potentially important new role in higher-pressure cases where CO2 supply is limited or unavailable.
The enormous increase in ethane production from shale plays, in concert with expanding ethane pipeline infrastructure, is creating new opportunities to leverage plentiful and low-cost ethane in higher-value applications such as recovering a portion of the billions of barrels of incremental oil that remain in mature onshore U.S. fields.
Editor’s Note: For more detailed information on ethane EOR technology, see SPE 179565, a technical paper the authors presented at the Society of Petroleum Engineers’ 2016 Improved Oil Recovery Conference, held April 11-13 in Tulsa. The authors acknowledge Russell Johns at Pennsylvania State University’s Earth and Mineral Sciences Energy Institute for providing the PennPVT™ tool kit used in the analytical MMP calculations.
PATRICK L. MCGUIRE is a senior consultant, reservoir engineering, at International Reservoir Technologies Inc. A former Society of Petroleum Engineers distinguished lecturer, he has designed and implemented large-scale EOR projects around the world. McGuire previously served in engineering, advisory and research roles at Maersk Oil & Gas, TNK/BP, BP Exploration (Alaska), ARCO Oil & Gas, Sandia National Laboratory and Los Alamos National Laboratory. He holds a B.S. in mechanical engineering from New Mexico State University and an M.S. in mechanical engineering from the University of New Mexico.
RYOSUKE OKUNO is an assistant professor of petroleum and geosystems engineering at the University of Texas at Austin. His research areas include phase behavior, thermodynamics, EOR, heavy oil recovery, reservoir simulator development, and unconventional resources. Okuno previously served as an assistant professor of petroleum engineering at the University of Alberta. He holds a bachelors and a masters in geosystem engineering from the University of Tokyo, and a Ph.D. in petroleum engineering from the University of Texas.
THOMAS L. GOULD is a senior engineering consultant and board member of International Reservoir Technologies. He is a recipient of the SPE’s Cedrick K. Ferguson Medal and is a former distinguished lecturer. With 46 years of industry experience, Gould has conducted numerous oil, gas and gas storage reservoir simulation studies, and has specialized in integrated studies management, thermal recovery simulation, and EOR simulation. He holds a B.S. in chemical engineering from Oregon State University, and an M.S. and Ph.D. in chemical engineering from the University of Michigan.
LARRY W. LAKE holds the Shahid and Sharon Ullah endowed chair in petroleum and geosystems engineering at the University of Texas at Austin. His research areas include integrated reservoir characterization, geostatistics, reservoir engineering, and EOR. A recipient of the Society of Petroleum Engineers Distinguished Service Award and a member of the National Academy of Engineering, Lake is a past SPE distinguished lecturer. He holds a B.S. in chemical engineering from Arizona State University and a Ph.D. in chemical engineering from Rice University.
For other great articles about exploration, drilling, completions and production, subscribe to The American Oil & Gas Reporter and bookmark www.aogr.com.