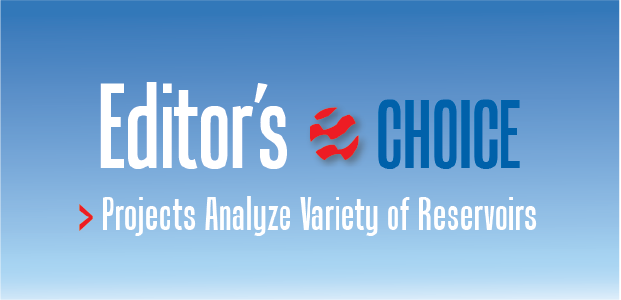
Projects Analyze Variety Of Reservoirs
By Kari Johnson, Special Correspondent
In the rolling plains of western Colorado, a team of researchers is exploring and mapping outcrops in an effort to better understand analogous formations deep underground. In a university laboratory, samples are evaluated for permeability, porosity and other petrophysical properties to better understand the rock matrix in shale plays. Elsewhere, scientists are investigating reservoirs to better understand the changes in the reservoir complex during carbon dioxide enhanced oil recovery operations.
Research projects conducted by universities and industry consortiums continue to advance the science of reservoir characterization and provide practical assistance to the industry. Whether designed to answer specific field-related questions or add to the general body of knowledge, these university-led projects are at the leading edge of reservoir characterization and modeling technology.
For 25 years, the Research Characterization Project (RCP) at the Colorado School of Mines has been working with the industry to characterize reservoirs with the goal of improving overall production. Much of its recent effort is devoted to CO2-based EOR and sequestration, according to Tom Davis, professor of geophysics and RCP director.
“Our objective is to bring together geology, geophysics and petroleum engineering in an integrated approach,” he says. “We deploy advanced technology to determine where CO2 moves within the reservoir and then use this knowledge to optimize sweep efficiency and oil recovery. The epitome of accomplishment for us is a strong increase in daily production.”
As an example of the potentially powerful combination of focused research and integrated geology, geophysics and petroleum engineering technology, Davis points to one field where CO2 flooding has transformed production economics, with daily production rates increasing from 10 to 3,000 barrels.
Over the past 15 years, RCP has conducted CO2 flood monitoring in the Permian, Williston, Anadarko, and Northeast Louisiana Salt basins. “After primary extraction and waterflooding, 70-75 percent of the original oil in place still remains in most fields,” Davis states. “Recovering another 15-20 percent by monitoring CO2 can add a lot of oil.”
The earlier that reservoir characterization work is performed in the life of a field, the more effective and economic tertiary recovery generally will be, Davis says. Once CO2 flooding has been initiated, detailed and regularly updated reservoir models can show where fluids are migrating and how to optimize injection well patterns.
According to Davis, the RCP also is conducting research in the oil-rich Bakken Shale play in the Williston Basin, with the emphasis on finding the sweet spots within the reservoir and determining how much the horizontal bore holes are contacting the reservoir.
“The goal is to develop methodologies that make it more likely that wells are placed in the sweetest spots to maximize production and recovery,” he remarks. “Multistage hydraulic fracturing opens fractures to allow the fluids to flow through the tight rock, and targeted placement of both horizontal laterals and fracs for maximum reservoir contact is key to efficient recovery.”
Operated by Whiting Petroleum Corp., the Postle Field in the Oklahoma Panhandle has been on production for nearly 50 years and still has an estimated two-thirds of its original oil in place. Davis and his team are working to characterize the field to optimize CO2 EOR. As part of the project, the team is mapping the CO2 flow over time through 4-D time-lapse seismic monitoring. “The rewards of characterization are two-fold,” Davis says. “It helps producers know how to optimize their EOR program and it also provides insights into CO2 trapping that can be used in carbon dioxide sequestration efforts.”
Studying the movement of injected CO2 and how it contacts and displaces oil has revealed a great deal of complexity, Davis states, noting that key learnings about various carbon dioxide phase characteristics are reflected back into RCP field projects.
“One particularly interesting area to me is using CO2 to optimize the performance of shale reservoirs,” he relates. “Although initially the emphasis in these fields is ‘frac-and-crack,’ there is an open question about what to do after the primary production phase is complete. Waterflooding may not be a good option because shales tend to swell when contacting water. Carbon dioxide or nitrogen may provide the best answer for what comes next after primary recovery in shale fields.”
Outcrops As Analogs
Many University of Colorado at Boulder students have enjoyed the outdoors while measuring, mapping and analyzing outcrops in northwestern Colorado. These outcrops, remnants of ancient river deposits, are visible versions of fluvial rivers now buried deep within the earth, notes Matt Pranter, associate professor of geological studies and director of the Reservoir Characterization and Modeling Laboratory (RCML) at the University of Colorado.
Using sophisticated measuring tools and old-fashioned legwork, project teams have put together impressive descriptions of rock and stratigraphy that can be used as parameters in modeling reservoirs thousands of feet below the surface. “Our goal in these outcrop studies is to assess the dominant controls on reservoir quality to more accurately map and model the spatial distribution of reservoir properties,” he explains.
While well logs and seismic data contribute invaluable information for reservoir characterization, they only can yield a partial picture because of the spatial sampling of the measurements. “Our teams augment these data with rock outcrop formation data,” Pranter remarks.
For the past seven years, researchers at RCML have been analyzing the Piceance Basin in northwestern Colorado. It is an ideal outdoor laboratory, according to Pranter, because the same formations that produce natural gas in the center of the basin are exposed at the surface along the perimeter. The multiphase project is funded by the Williams Fork Consortium, a group of companies interested in natural gas development associated with the fluvial deposits. The team has been working on the western and southwestern rim of the basin out near Grand Junction in canyons where rocks are exposed and accessible. The nearest significant natural gas fields are 25-50 miles away, he says.
This kind of study can provide valuable feedback to the industry. For example, Pranter explains that one study by a professor at Mesa State University was used by a Denver-based independent to justify decreasing well spacing from 20 to 10 acres. “The study essentially showed that the width of the targeted reservoirs was likely smaller than the well spacing,” he comments.
Dimensional statistics from outcrops and deposits can be combined with subsurface detail in reservoir models. “While there are no substitutes for seismic and well log data, physical measurements of the outcrops can be crucial. The statistics effectively constrain the reservoir model. Information on how bodies change with depth and stratigraphy also can be used as constraints,” Pranter points out, noting that porosity and permeability are not a main focus of the outcrop study because the rocks have been exposed over time at the surface, and therefore, may not be good analogs for the rocks buried in the subsurface.
Typically, he says RCML investigators study subseismic detail. The dimensions of fluvial reservoirs can be 10-30 feet thick and 300-400 feet wide, which generally fall below the resolution of conventional 3-D seismic in the Piceance Basin.
Grand Valley Field Study
Now in its fifth phase, the RCML is focused on detailed modeling at the Grand Valley Field north of Parachute, Co. This is an expanded analysis of earlier work from north of Palisade, Co., to include the Douglas Creek Arch south of Rangely, Pranter details. “We are gathering data from numerous measured sections. In this interval, we describe the rock foot by foot or even centimeter by centimeter in terms of rock type, grain size and sedimentary characteristics,” he remarks.
At the same time, outcrop gamma-ray logs are created as the equivalent to well-based gamma ray logs. Using a gamma-ray scintilometer, the natural radiation of the outcrop also can be compared to the gamma-ray logs from wells, according to Pranter.
RCML researchers also have acquired terrestrial and aerial light detection and ranging (LIDAR) data, Pranter reveals. The terrestrial data provides detailed elevation data, while the high-resolution aerial LIDAR and orthophotographs of 65 square miles were used to obtain additional positional and dimensional information. “These data were used to supplement the data collected by walking the field with a hand-held GPS for those areas not easily accessible on foot,” he explains. “The data will be used in 3-D modeling of deposits in the subsurface to constrain the bodies to honor the histogram of thicknesses or width based on outcrop.”
The Department of Energy’s National Energy Technology Laboratory is sponsoring a new project that focuses on the challenge of upscaling reservoir properties such as permeability and porosity, according to Pranter. Models are divided into cells, each of which may be several feet thick, with one value for permeability and porosity assigned to the cell. At the same time, core data may provide detail in increments as small as one inch.
“Reconciling these data in a way that produces a representative model is a significant challenge,” Pranter holds. “We would like to find ways to upscale, ideally based on the geology or facies involved, rather than a single mathematical transform without geological constraints.”
The project involves the Dakota formation of the Denver Basin and has implications for CO2 flooding and sequestration as it seeks to better understand and estimate pore volume, pore volume distribution and how pores may leak or seal. This, ultimately, indicates the size of the reservoir for extraction, or how much storage space is available for sequestration, Pranter says.
Another permeability and porosity study is starting in collaboration with the Colorado School of Mines. Similar to the Williams Fork Consortium, researchers will study permeability, porosity and other characteristics of the Mancos/Niobrara tight oil/gas formation, Pranter reports. The specific goals of the project are still in development, but he says the work is certain to include detailed modeling using field production data, stratigraphy and structure to develop geological controls on reservoir performance.
Multicomponent Seismic
The Bureau of Economic Geology in the Jackson School of Geoscience at the University of Texas at Austin is focusing on applying multicomponent seismic to improve the understanding of fracture systems. The Exploration Geophysics Laboratory (EGL) within the BEG is researching on acquiring, processing and interpreting both compressional (p) and shear (s) waves because studies have shown s-waves to be more sensitive to fractures than p-waves, says Bob Hardage, senior research scientist at the EGL.
“It is absolutely necessary to understand fracture systems to optimize production in unconventional fields,” Hardage claims. “Multicomponent seismic technology contributes to that understanding by increasing the number of attributes that react to rock properties, because combining p- and s-wave data yields more attributes that can help define rock and fluid properties.”
The BEG is conducting studies in the Marcellus, Eagle Ford, Barnett and other shales, as well as tight gas sandstones. It maintains 10-12 industry consortia in several disciplines, including the EGL. Hardage says an important factor in these projects is integrating multiple disciplines, including geophysics, geology, rock physics, geochemistry and engineering.
One project, funded by the DOE, focuses on demonstrating the value of multicomponent seismic for characterizing porous brine reservoirs as potential CO2 sequestration targets. The study is focused on the Midwest region, but extends into Pennsylvania, where Hardage says 3-D multicomponent data have been acquired in porous brine reservoirs.
“The analysis incorporates all available field data, including bore hole seismic, surface seismic, well log data and anything else that can tell us about rock and fluid properties,” he remarks. “S- and p-wave data have different reflectivities at rock interfaces. At every site we have studied, there have been interfaces that the p-waves detect and others that the s-waves detect.”
And that, he says, is why both components are needed. “They react to different elastic properties and rock layering,” Hardage remarks. “We are trying to show that the two images of p- and s-waves are simultaneously different, and yet both images are correct.”
The multicomponent seismic data are inverted to derive seismic amplitude and elastic properties. It is critical to understand the rock and fluid properties to know why the images are different, Hardage goes on. “That is why, in everything we do, one of the most fundamental things that has to go along with our seismic research is rock physics research that explains how rock and fluid properties influence p- and s-wave reflectivity.”
In unconventional plays, Hardage claims that multicomponent seismic technology also provides special insights. “As shear energy propagates through rock layering and encounters a fracture system, it undergoes wave splitting,” he says. “The shear wave separates into fast and slow-mode waves. The fast mode has its particle displacement vector oriented parallel to fractures, while the slow mode is perpendicular. P-wave does not have this separation.”
Shear waves also exhibit a phenomenon that is indicative of the presence of fractures, Hardage adds. The challenge is to recognize this splitting and extract the fast and slow modes from the data so that a 3-D volume can be made of each set of data.
The azimuth in which the fast mode is oriented implies the azimuth of the fracture, he notes. The relative speed of the slow mode indicates relative intensity; the slower the seismic wave, the greater the fracture intensity. “With this orientation and intensity information, oil and gas companies can know where to place horizontal well bores and calculate horizontal stress values to indicate the likely paths that hydraulic fractures will take away from the well bore,” Hardage states.
CO2 Sequestration
The Gulf Coast Carbon Center (GCCC) within the BEG is collaborating with partners in 24 universities, six national laboratories and the U.S. Geological Survey, plus other groups within the University of Texas, all funded by DOE and a dozen industry sponsors to test the feasibility and best practices for injecting CO2 into deep geologic formations. The team is working with three brine reservoirs and two EOR sites (Figure 1) to confirm that sequestration is safe and effective, reports Susan Hovorka, senior research scientist at the GCCC. A key goal is to ensure that CO2 does not get into the atmosphere or migrate to freshwater aquifers.
“Our research is a bit of the reverse of production in that we inject into rather extract from the reservoir,” Hovorka notes. “To be successful at sequestering CO2, we must understand as much as possible about the reservoir. To do so, we use essentially the same reservoir characterization methods used for production.”
An interesting side effect of carbon dioxide sequestration research is its positive impact on reservoir characterization techniques, says Hovorka. “A considerable effort is devoted to tracking what happens to CO2 after it is injected, which in effect, tests the validity of the characterization,” she comments. “Improvements made as a result of this information can benefit EOR and reservoir characterization projects.”
Retention must be high for CO2 sequestration to be successful. “In EOR, retention is simply measured in barrels. but we need more precision for sequestration and we must ensure that trapping lasts for hundreds of years versus the operational life of a producing field,” Hovorka points out.
Projects use classic reservoir characterization techniques combined with state-of-the-art geophysical technology, she goes on. Carbon dioxide is injected and tracked using reservoir pressure data, production data, 4-D surface seismic, downhole seismic, crosswell seismic and vertical seismic profiling and well logging (Figure 2). The team also uses a deep-resistivity logging array and an intensive tracer program to look for changes in travel time from one well to another to understand how much of the pores are being contacted under different injection rates. Through this process, Hovorka says the team determined that increased injection rate leads to improved sweep efficiency.
As part of the reservoir characterization work, GCCC is testing several fundamental conceptualizations. It has found that its conceptualizations of residual water saturation and residual CO2 sequestration were valid, and its buoyancy conceptualization is still under review, according to Hovorka. Unlike oil and gas, where the fluid is up against a seal, injected CO2 comes with energy or gradient that interacts with buoyancy. “Which is more important is a very big theoretical question,” says Hovorka.
The team is in the middle of history matching the characterization. “We are looking at how many characterizations we can history match to see how good the characterization is, especially with regard to sweep efficiency,” she details. “So far we have quite respectable matches.”
Repeat crosswell seismic, VSP and surface seismic are scheduled for the fall. “Then we will see how the reservoir responds to CO2 in a heterogeneous fluvial reservoir,” Hovorka continues. “We have already seen the results of the tracer tests, which were fascinating. We see the tracers coming through at multiple breakthroughs and different flow paths developed over time. Once established, we thought they might maintain the same flow paths, but as we inject at higher flow rates, we are seeing CO2 accesses more of the pore volume. As a result, we are investing more in relative permeability and capillary entry pressure testing of the cores to history match for increasingly sophisticated characterization.”
EOR operators generally assume that the CO2 goes updip and accesses the reservoir, and then migrates toward production wells. Hovorka says GCCC will make another set of measurements in the coming months to look at different scenarios for how to conceptualize the interactions of different types of physics on multiphase fluids in a complex flow system. The goal is to provide insight into how to characterize multiphase flow in all kinds of reservoirs, including those that have oil and gas, she adds.
Other questions remain that could have implications for EOR, including the dissolution of carbon dioxide into water, the adequacy of well completions at retaining injected CO2, the effects of pressure increase on sealing faults, the effective management of downdip migration, detecting fugitive CO2 and documenting plume edges, according to Hovorka.
“The value of our research goes beyond storing captured CO2,” she concludes. “The only way we can do more widespread EOR is to tap into anthropogenic sources of carbon dioxide, which only can be done if we can show that the CO2 is being retained in the reservoir. Reservoir characterization is fundamental to demonstrating retention.”
Improving Production
At the Institute for Reservoir Characterization (IRC) at the University of Oklahoma, investigators are seeking to increase oil and gas production in shales, deep water and more traditional reservoirs. “One of the big values of reservoir characterization is that it will normally lead to improved production,” notes Director Roger Slatt. “Studies show that two-thirds of the world’s production comes from mature fields that have been producing for more than 25 years. Even a 10 percent improvement in overall production would add 9 million barrels a day.”
About half of the IRC’s 20 students are working on projects to leverage reservoir characterization to improve production in shales. Initial projects were exclusively in gas shales, but Slatt says projects are beginning to look at oil and gas liquids shales. A goal is to identify the laterally continuous extent and rock type of the subsurface horizon for optimal well bore placement and artificial fracturing, he notes.
The study of shale geology and its integration with seismic, geochemistry, engineering, rock physics and geomechanics is relatively new, Slatt points out, and there is still much to learn through reservoir characterization that can impact drilling and completion economics. “Traditionally, shales were thought to be uniform from top to bottom,” he says. “We now know that the stratigraphy or layering of shales is quite complex and some horizons are definitely better for production than others. One important area for research is in improving well logging tools such as borehole imaging for shale reservoirs because they are so highly stratified. Most conventional logging tools cannot resolve the fine details required.”
Adding to the issue are uncertainties in accurately measuring shale permeability, porosity, and fluid flow. “The techniques used today were developed for sandstones and carbonates,” Slatt explains. “New methods specific to shales could improve reserve estimates and production planning.”
Shales are fine-grained, changing the properties of flow. “Particles in shales are the size of the tip of a pin rather than of the pinhead-sized grains in sand,” Slatt describes. “When you put pinpoints together, there just is not as much space for the fluid to flow. Even if a shale formation contains a lot of hydrocarbons, the trick is to get the hydrocarbons to flow at economic rates. This typically requires artificial fracturing of the formation.”
Knowing the extent of a laterally continuous horizon of good, fracturable shale is key to optimizing production, he goes on, noting that the features that control production are often at the subseismic scale, beneath conventional seismic resolution. Research projects to improve that resolution require tight integration between geology and geophysics, he says.
Slatt points to one project that analyzed core-calibrated well log responses for regional interpretation and correlation of different rock types in uncored wells within a shale reservoir. The technique has proven applicable to other shales, provided improved statigraphic interpretation for predicting distribution of brittle and ductile rock types at different scales (thicknesses), as shown in Figure 3.
In this figure, the first column is a gamma ray log of reservoir, the second column is a borehole image log of part of the reservoir, the third column is a core description (with each color representing a different rock type), the fourth column shows brittle (green)-ductile (red) coupling at the sequence (reservoir) scale, while the fifth and sixth columns panels show couplets at the parasequence and bed-set scales, respectively.
Other studies focus on the stratigraphic aspects of shales and the effect of fabric, porosity and composition on geomechanical properties at the reservoir to the pore scales. Figure 4 shows the variety of pin (micron)-sized pore types that occur in shales. The white bar scales in each image are 1-2 microns in length.
IRC tight gas research is focused on the Jackfork Group of Arkansas and eastern Oklahoma, and the Lewis Shale in Wyoming. Students have measured and interpreted a near-continuous 2,000-foot Jackfork section and conducted a geologic comparison to deepwater reservoirs worldwide. The study of such tight gas sands has been a central research focus for the Institute in recent years, according to Slatt.
Included in this research is the 3-D characterization and modeling of outcrops, then inputting them into reservoir simulators for fluid flow simulation. The example in Figure 5 shows the results of simulating the “reservoir performance” of a deepwater Jackfork outcrop that is analogous to deepwater reservoirs in the U.S. Gulf of Mexico. Slatt says the benefit is being able to see the rock characteristics in the outcrop to help explain reservoir production performance.
Slatt developed an integrated systematic workflow designed to optimize the measurement and interpretation for tight gas sand and shale reservoir characterization. The workflow incorporates core and outcrop lithology and sequence stratigraphy, geochemistry, petrophysics, geomechanics, well log and 3-D seismic analysis. “Integrating these techniques into a 3-D geocellular model allows for documenting and understanding the fine-scale stratigraphy of shales and tight gas sands and provides input into reservoir performance simulators as an aid to improved horizontal well placement,” he concludes.
For other great articles about exploration, drilling, completions and production, subscribe to The American Oil & Gas Reporter and bookmark www.aogr.com.