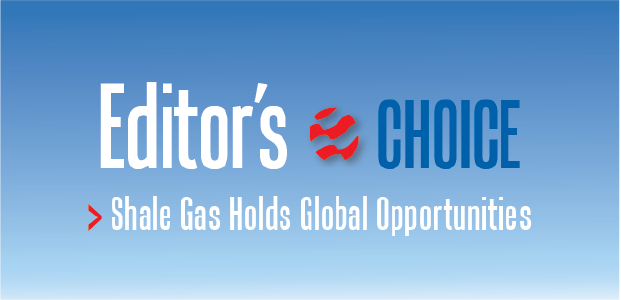
Shale Gas Holds Global Opportunities
By Stephen A. Holditch
COLLEGE STATION, TX.–From the Barnett Shale–which the U.S. Energy Information Administration ranks as the most productive gas field in the nation with some 14,000 wells producing more than 5 billion cubic feet a day in 2009–to the Bakken–which the U.S. Geological Survey pegs as the largest continuous oil accumulation it has ever assessed–to the Marcellus–which even in its infancy is being recognized as one of the biggest gas deposits on the planet with an estimated 500 trillion cubic feet of recoverable reserves–everybody in the world is looking at drilling and completion technologies to optimize the development of shale reservoirs.
I began giving presentations at technical meetings in the late 1990s on the role of unconventional resources in the future worldwide supply of oil and gas. The main point was that the in-place volumes of unconventional gas and heavy oil were enormous compared with conventional proved oil and gas reserves. At the time, unconventional gas was only a blip on the radar screen. Today, it accounts for almost half of all natural gas produced in the United States.
It was clear to me then that there was plenty of oil and gas in unconventional reservoirs, as long as the economics and technologies were in place to allow the industry to efficiently develop them. What I never imagined, however, was how quickly and dramatically unconventional gas would impact the North American market. No one could have predicted 10 years ago the incredible growth in U.S. gas supply from unconventional formations (especially shales), and volumes continue to increase every year. Within another 10 years, unconventional gas is expected to represent two-thirds of gas production in the United States.
Shale plays have been the main driver in the growth of unconventional gas supply, beginning with the development of the Barnett and then quickly expanding into the Woodford-Caney, Fayetteville, Marcellus, Haynesville and Eagle Ford. According to the U.S. Department of Energy, U.S. shale gas production was expected to increase by almost fourfold between 2007 and 2020, with the potential to sustain high production output for decades to come.
Two “breakthrough” technologies have reshaped the economic profile of developing gas shale: horizontal drilling and multistage hydraulic fracturing. I participated in projects in the 1980s to develop the Ohio Shale in the Appalachian Basin and the Antrim Shale in Michigan using vertical wells and foam fracturing treatments to avoid damaging the low-pressure shales. Creating long, conductive fractures was a major struggle in those early efforts. The game changed with the introduction of horizontal drilling and water frac techniques, which date to the pioneering efforts of Union Pacific Resources Company in the Austin Chalk in the early 1990s. In fact, the Austin Chalk is the model for modern shale development methods. Applying the same basic techniques developed in the Chalk, operators have opened the shale frontier–from the Barnett to the Bakken.
Going forward, the biggest technological benefits likely will be found in incremental improvements designed to lower costs. At today’s wellhead prices, the key to making money in gas shales is controlling cost, which is probably true in most oil and gas operations most of the time. Better bits, drilling fluids, fracturing fluids, proppants, etc., that enable wells to be drilled and completed at lower overall cost are probably the biggest areas of need for the foreseeable future.
Estimating Reserves Recovery
Historically, one of the challenges in shale plays has been the ability to accurately estimate how much gas a well will recover over its life cycle. Simply put, recovery volumes in gas shales depend on the length and width of the reservoir, which are controlled directly by the geometry of the horizontal well and the hydraulic fracture stimulation. The length of the lateral and the stimulation treatment’s effectiveness at fracturing the rock determine the reservoir volume effectively drained by the well, which in turn, dictates ultimate recovery potential.
The width of the drainage area is a strong function of the design and quality of the stimulation treatment. If a small treatment is pumped that does not crack much rock, the well will drain a long and narrow rectangular volume of rock along the path of the well. On the other hand, a large treatment that pumps more fluid and proppant creates more fractures to connect more of the reservoir to the well bore, creating a wider and squarer-shaped rectangular volume.
Fluid volumes and injection rates/pressures are certainly part of the equation of an effective fracture treatment, but the main component is the amount of proppant placed in the created fractures, whether it is Ottawa sand, resin-coated sand or ceramic proppant. The goal of any fracture treatment in a gas shale should be to put away proppant to keep fractures open after the rock has been cracked to allow gas to flow to the bore hole. The more fluid and proppant pumped, the greater the drainage volume likely will be.
During the process of fracture treating a horizontal well starting from the tip of the lateral and working toward the heel, microseismic measurements give the operator a rough idea of the volume of rock being stimulated. Estimating the total volume of the reservoir area that will be drained by the well is a fairly simple process of multiplying the length of the lateral by the width of the stimulated area and the thickness of the reservoir.
It also is possible to estimate the recovery factor by correlating porosity with permeability measurements from core data. It is clear that porosity matters in shale plays. Porosity in the sweetest parts of the Haynesville Shale is 14-16 percent, and the better parts of the Eagle Ford have 12-15 percent porosity. By contrast, less prolific shale formations have a porosity range of 6-10 percent or less.
Porosity not only helps control the amount of gas in place in the pore spaces, but in general, the more porous the zone, the more permeable it becomes. Consequently, a relatively small number of wells drilled in areas with the best porosity and permeability may produce a large portion of the total gas in place in a shale play. Outside the high-porosity/high-permeability areas, a much larger number of the wells will have to be drilled to produce an equivalent volume of gas.
By determining the volume of rock that a multistage fracture treatment connects to the lateral, and factoring in porosity and permeability measurements, it is possible to get a good preliminary estimate of how much gas can be produced. Obviously, once a well comes on stream, production data and decline curve analysis can determine actual reserves and ultimate recovery values (similar to a conventional reservoir). However, the quality of the fracture treatment is critical in shale plays because it determines the size of drainage volume connected to the lateral, and therefore, how much gas it can produce.
Well Design Limitations
From the earliest phases of horizontal development in the Austin Chalk and then the Barnett Shale, the secret to success has been hooking up the vertical extent of the reservoir to the horizontal bore hole using hydraulic fracturing to create gas flow pathways. Without multistage fracture treatments to break the rock and keep the fractures open, a horizontal well would produce little gas no matter how far the lateral extended through the formation.
If the primary factors controlling productivity and recoverability are the width and length of the stimulated volume of rock, theoretically, a well should become more economic the longer the lateral becomes and the more fracture stages are pumped. While that may be true on a computer screen, real-life cost and risk scenarios impose practical limitations on lateral length and number of fracture stages per lateral.
Cost issues aside, the undeniable fact is that mechanical risk increases proportionately with lateral length and number of stages. Each stage of a hydraulic fracture treatment cools the casing to essentially the temperature of the fracture fluid at the surface (typically 70-80 degrees Fahrenheit) all the way to the bottom of the hole, which causes the casing to shrink as a result of ballooning forces and the temperature change. A 10,000-foot string of pipe will shrink tens of feet as it cools. When pumping stops, pressure on the casing string decreases and the pipe then begins to heat and expand. These repeated shrink/expand cycles put enormous stress on the casing string and can cause integrity issues.
While 10-20 or more stages are commonly pumped in some wells, others have experienced casing failures after as few as five stages. Because fracturing stages begin at the toe of the lateral and work toward the heel, casing failures tend to occur during the latter part of treatment near the horizontal turn, which means the entire lateral section is jeopardized. Putting heavyweight pipe in the heel area can help overcome this problem, but at some point, the risk profile and cost-to-benefit ratio will limit well length and number of stages.
The industry is very good at finding the right answer through trial and error, and it appears that 4,000-6,000 feet is the optimal lateral length in shales with five to 10 frac stages. Although laterals of twice that distance and with three times more frac stages have been successfully drilled and completed in the Bakken and other formations, the risk involved in engineering ultralong laterals with 20-30 frac stages will generally outweigh the potential benefits in most shale plays.
Shale Gas Economics
When I began giving the lecture on unconventional resources 12 years ago, one of the requirements to exploiting this supply base was higher commodity prices to support the increased associated finding and development costs. Fortunately, a strong gas price incentive was in place throughout much of the past decade, giving oil and gas companies ample opportunity to experiment in various shales. Ironically, as commodity prices weakened in 2009, shale gas emerged as the low-cost supply source in North America, with conventional gas fields relegated to higher-cost status.
Much has been made of “break even” price thresholds in shale plays, but there is no one gas price at which a particular play becomes economic. All shales, including highly prolific formations such as the Haynesville, have relatively confined core areas where geologic characteristics are most favorable. In some plays, a $3 or $4 an Mcf gas price may be sufficient in the core area to generate a return. But as drilling moves outside the core, the shale thickness, porosity and permeability change, and wells with identical lateral lengths and fracturing stages produce less gas, requiring a higher price to make the economics work.
As in the Barnett, the industry will find the full economic spectrum in shale plays, with some areas where a reasonable return on the investment can be made at one price, and other areas where the same price is inadequate because well performance is poorer. This spectrum of higher- and lower-quality areas is true of any unconventional gas reservoir anywhere, and the lower-quality areas (likely representing the largest collective part of a play) require either a higher price or a lower development cost.
As part of a Texas A&M research project, graduate students are analyzing the Barnett Shale economics, specifically looking at percentages of the play that are economic as a function of gas price, which is also a function of finding and developments costs. If F&D costs can be reduced, a larger percentage of any shale play will be economic at any given gas price.
Initial year decline rates reported as high as 80-plus percent is one of the factors impacting shale gas development economics. In the Haynesville and Eagle Ford, for example, wells come in making initial rates of 10 million-30 million cubic feet a day, but then quickly decline to 4 MMcf-6 MMcf a day. In these wells, reservoir pressure can be pulled down to pipeline pressure within only 60 days. To lower such steep decline rates, I advocate pinching back flow rates with smaller chokes during the first six to 12 months of production.
It has been my observation over the years in deep, high-pressured gas wells–especially those that are overpressured, as is the case in parts of the Haynesville and Eagle Ford–that using big chokes to facilitate high flow rates rapidly draws down pressures, which can cause permanent damage to the reservoir as well as the hydraulic fractures around the well bore. Moreover, both the Haynesville and Eagle Ford are geopressured formations that have not been compacted in certain areas, which means reservoir permeability declines as pressure is reduced. Of course, once damage has been done to a formation or completion, it cannot be reversed by pinching back the well.
Using smaller chokes to maintain production rates of 5 MMcf-10 MMcf a day allows the reservoir pressure near the well bore to decline slowly over a period of months. Productivity and flowing tubing pressure still decrease in time, but less damage is done to the reservoir and the fracture system, and in the end, the wells should produce more gas and recover more reserves.
Global Opportunity
The United States has led the way in developing shale reservoirs, and there is a tremendous opportunity to export the technology and expertise to plays around the world–not only for independent operators, but also for service companies. In fact, several of the independents who have pioneered the U.S. shale plays have already scoured the globe for basins where they can repeat the North American shale gas success story. There are hundreds of basins that contain shale, but the easy part is finding prospective shales. The hard part is accessing markets to take the produced gas and finding personnel capable of executing the technology in the field to drill and complete the wells.
The world is full of stranded deposits of conventional gas that were found by chance while searching for oil, and gas without a market has little value. A company can find the best gas shale in the world, but if it is located a long way from market infrastructure, making money on the discovery is going to be very difficult. To have value, gas shales need to be located near regions with established markets and pipeline access.
The other problem is a familiar one for oil and gas companies that do business overseas: making certain the right team is in place to do the work. Technology can be exported anywhere, but it takes a special crew with the proper training to operate the rig and downhole equipment needed to drill a 5,000-foot lateral 12,000 feet under the ground. It also takes a special group of people with specific skills to put out a frac spread and spend four or more days on location working around the clock to stimulate a well in multiple stages. A company can do everything else right, and it will lose money if the fracture treatment is not executed flawlessly.
Of all the shale basins around the globe, only a small percentage today has the local market infrastructure and service company capacity to make investing in shale gas worthwhile. A good example is Europe, which is similar to North America in that natural gas is used both to generate electricity and for heating during the winter. There are a lot of shales in Europe, and European nations are trying to be proactive by developing shales to take control of their own natural gas supply destinies. Based on the way sedimentary rocks are deposited, odds are that the more Europe is explored, the more shale gas and other unconventional resources will be found.
For the time being, the global opportunity in shale gas is limited to select areas where markets and service expertise exist, but one day there will be Barnett, Haynesville and Eagle Ford look-alikes all over the world. I do consulting work with several companies in the Middle East, and recognizing that conventional hydrocarbon volumes in the Middle East are so vast, it is possible to conclude that the region’s volumes of unconventional resources could be gigantic. I never thought I would see the day when the industry would look for unconventional resources in the Middle East, but that is precisely what is happening.
From Europe to the Middle East, Australia and South America, shale gas is going to become as big a deal around the globe over the next 20 to 30 years as it has become in North America over the past 10 years.
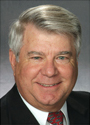
STEPHEN A. HOLDITCH is the department head and Samuel Roberts Noble Foundation Endowed Chair in petroleum engineering at Texas A&M University. He began his career as a production engineer at Shell Oil Company and joined the Texas A&M faculty in 1976. One year later, he formed S. A. Holditch & Associates Inc., serving as president and chief executive officer of the petroleum engineering consulting company, which was acquired by Schlumberger in 1997. He was named a Schlumberger Fellow in production and reservoir engineering in 1999. Holditch is an honorary member of both the Society of Petroleum Engineers and the American Institute for Mining, Metallurgical & Petroleum Engineers, the highest awards given by the societies. He is also a past SPE president, distinguished member and board member, and a former AIME trustee. He has been elected to the U. S. National Academy of Engineering, the Russian Academy of Natural Sciences, and the Texas A&M Petroleum Engineering Academy of Distinguished Graduates. Among his professional honors, Holditch is the recipient of SPE’s Lester C. Uren and John Franklin Carll awards, the American Society of Mechanical Engineers’ Rhodes Industry Leadership Award, and the SPE/AIME Anthony F. Lucas Gold Medal.
For other great articles about exploration, drilling, completions and production, subscribe to The American Oil & Gas Reporter and bookmark www.aogr.com.